From Design Copying to Mathematics in the Early Childhood Classroom
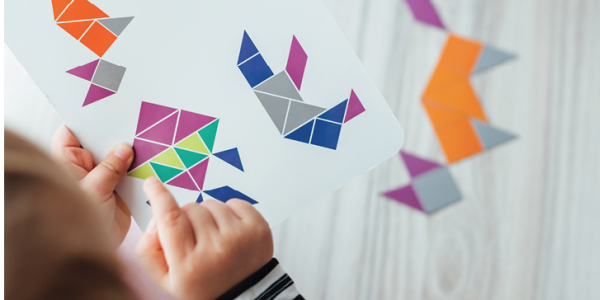
You are here
As a kindergarten teacher, I received glimpses of my future every February during kindergarten registration. While other members of the school staff helped parents fill out forms, I conducted the state-mandated screening of my soon-to-be students, many of them still wide-eyed 4-year-olds more accustomed to pretend play than formal assessments. During the screening, I asked them to identify and describe various objects (a ball, a button, a car), stand on one foot, skip down the hallway, draw a person, and copy four simple shapes. Most children thought we were just playing games, but a few would fix me with a quizzical look as if to say, “What kind of teacher doesn’t know what a button is?” Truthfully, I agreed with the bemused kids; the screening seemed as odd and decontextualized to me as it did to them.
Most of the time, I would quickly calculate the child’s score, note that it was above the minimum cutoff, and tuck the recording sheet into a folder from which it never emerged again. I valued kindergarten screening because the one-on-one interaction allowed me to get a sense of my future students, but the outcomes of the assessments rarely concerned me.
Why should I care if a young child could copy a square? What could such a trivial task portend? Now, after reviewing the research, I see that design copying is an essential skill with powerful implications for how we cultivate young children’s knowledge and abilities in mathematics.
—Tony Byers
Indeed, we all might wonder what bearing a child’s ability to copy a square has on her mathematical development. Research, however, indicates that how well a 4-year-old can copy a relatively simple shape predicts later math performance. This skill, known among researchers as design copying, tends to be assessed in young children as one of many measures of fine motor skills. For example, in one nationally representative dataset called the Early Childhood Longitudinal Study of Kindergarten (ECLS-K), the fine motor composite comprises three tasks: building a bridge with blocks, drawing a person, and copying five designs. Studies with this dataset indicate that fine motor skills measured during kindergarten robustly predict math achievement in first, third, and fifth grades; and fine motor skills are as predictive of later academic achievement as teachers’ ratings of children’s attention (Grissmer et al. 2010; Murrah 2010). Another study suggests that this association between cognitive and motor development seems to be in place even before children enter kindergarten (Chen 2010). In datasets that allow for separate analyses of the different fine motor tasks, design copying tends to be the most predictive of later math achievement compared with other types of fine motor skills (Cameron et al. 2012; Carlson, Rowe, & Curby 2013).
Essential skills
What is so mathematical about a 4-year-old’s shaky rendering of a simple shape? The easiest way of thinking about the connection is to scale the task up to an adult skill level. While the majority of adults can easily copy a square, how many can do the same with a more complex figure, like a dodecahedron? Most adults have the fine motor skills to complete the challenge, but something else determines whether we produce a nice, crisp dodecahedron or a picture of a deflated soccer ball. Try to copy the dodecahedron shown. You’ll likely find yourself mentally breaking the figure into parts, shifting your attention between the model and the drawing, struggling to translate your mental image of the figure onto the page, monitoring your progress, and correcting your errors.
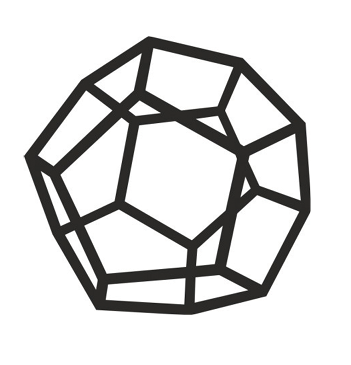
Copying tasks can be difficult for both children and adults because they rely on a wide range of spatial skills, such as assembling parts into a whole, comprehending spatial relationships, and visualizing objects from multiple perspectives (Uttal et al. 2013; Verdine et al. 2014). While our spatial ability is what allows us to understand what we see and to navigate the world, it is also connected to our ability to do math. A substantial body of research shows overlaps between areas of the brain that process spatial information and those that process numerical information, such as location and size (for an engaging technical review, see chapter 10 of Dehaene 2011). Brain areas that evolved to handle basic numerosity (like number sense) recruit nearby spatially oriented areas to assist with more complicated mathematical operations (like simple arithmetic). Accordingly, spatial skills are associated with general mathematics ability (Bull, Espy, & Wiebe 2008) and are often compromised in individuals with mathematics learning disabilities (Geary 2013). Further, high school students who do well on tests of spatial skills are more likely to pursue STEM careers than their lower-scoring peers (Wai, Lubinski, & Benbow 2009).
Activities that support mathematics achievement do not always look like math.
Of course, spatial skills are not solely responsible for how well a young child can copy a design. Design copying tasks also require executive function, a term that is increasingly familiar to early childhood educators. Executive function includes a diverse set of skills, including sustaining attention, shifting attention intentionally, controlling impulses, and holding information and procedures in working memory (Best & Miller 2010). Practically speaking, these are the cognitive skills that allow children to manage their thinking and behavior (McClelland & Cameron 2012). Children who have trouble stowing their belongings in their cubbies, who drift from one activity to another, and who cannot seem to remember simple multistep directions may be struggling with one or more components of executive function.
Both design copying and mathematics place similar demands on executive function. While copying, a child must plan the order in which he will replicate parts of the design and maintain a representation of the design and his plan in his working memory while he shifts his attention from the model to his drawing. While doing math, the demands are similar—and executive function is consistently associated with math performance, particularly during early elementary school (McClelland & Cameron 2012; Verdine et al. 2014).
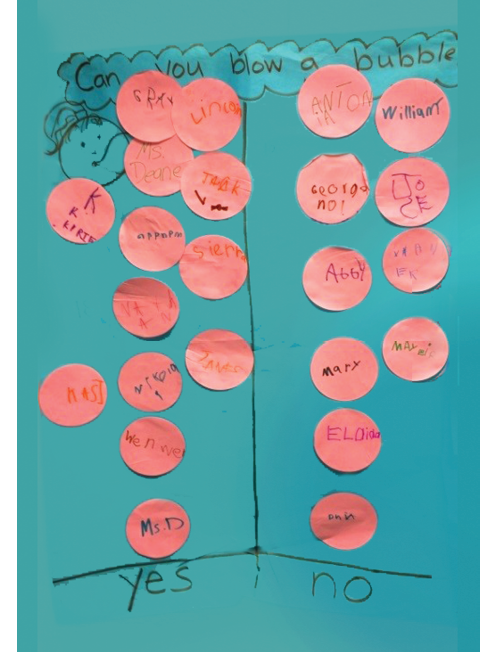
There are myriad ways in which executive function and spatial skills make their way into the kindergarten classroom, particularly during math instruction. In the K–2 wing of most elementary schools, the children’s work being displayed usually relies heavily on executive function and spatial skills to plan and create. Pictured is an example of one such product posted outside of a kindergarten classroom in Charlottesville, Virginia. In this lesson, the teacher supported the students in doing the following:
-
Think discriminately about the question, “Can you blow a bubble?,” separating extraneous from pertinent information (directing attention and controlling impulses)
-
Identify in which column to place the circles representing their opinions (working memory and spatial skills)
-
Draw or paste each circle on the butcher paper, using proper ordering and spacing (fine motor coordination and spatial skills)
-
Answer concluding questions, such as “Can your teacher blow a bubble?” and “Which is bigger—the number of children who can blow a bubble or the number who can’t?” about what can be learned by looking at the graph (working memory, shifting attention, processing spatial information)
Looking at math lessons through this lens, it is easy to see why children with strongly developed executive function and spatial skills develop mathematical ability more easily than their peers who are weaker in those areas.
Design copying and mathematics require a similar combination of cognitive skills, including executive function and spatial skills.
The connection, then, between design copying tasks and mathematics is that both require a similar combination of complex, interrelated cognitive skills, including executive function and spatial skills. For teachers, knowing this may open the door to a new kind of math instruction, one that does not seem to focus on math at all.
From theory to practice
Practically speaking, how might this insight be applied to classroom instruction? In the same way that speed cultivated on the track helps a football player on the field, the cognitive skills honed during design copying tasks may help children learn math. There is, however, a reasonable objection that early childhood educators may raise. Even the term design copying makes it sound like tedious work. Copying hundreds of figures resembles the kind of developmentally inappropriate activity that sometimes darkens the doorways of preschool and kindergarten classrooms across the country. Or does it?
Consider the familiar crafts and games that have been the staple of preschools, camps, afterschool programs, and lazy Saturday afternoons for decades. Materials like Play-Doh, LEGOs, yarn, Colorforms, fuse beads, and Wikki Stix offer countless hours of creative play—and they also afford children the opportunity to precisely recreate models, sometimes translating two-dimensional pictures into three-dimensional creations. What are young children doing when they assemble hundreds of tiny LEGO pieces into the pirate ship they see on the front of the box, if not design copying? A team of researchers, educators, and psychologists (including the authors of this article) used this insight to create and test a curriculum meant to improve early math skills using fun design-copying activities.
Minds in Motion
In an afterschool classroom, seven typically rambunctious kindergartners silently hunch over their fuse bead designs, carefully placing tiny beads onto even tinier posts. They are trying to copy designs their teacher matched to their skill levels. They pick up several beads at a time, count and sort them, and arrange them to match their models. As they work, their instructor monitors their progress and provides verbal cues, often laden with spatial language, such as “Does the yellow bead go above or below the orange bead?”
This kind of focused arts and crafts activity, familiar to many early childhood educators, is at the heart of the Minds in Motion curriculum (Brock et al. 2017). Minds in Motion is designed to complement and enhance motor and cognitive development between the ages of 4 and 7. Each lesson capitalizes on developmental milestones, such as the ability to copy geometric shapes, print letters, stack 10 or more blocks, recognize at least four colors, and follow two- and three-step directions (American Academy of Pediatrics 2009). Respecting the developmental variability of children, the program differentiates not only the processes by which children complete tasks, but also the specific designs they copy.
Minds in Motion uses materials and activities that are readily available in most preschool and kindergarten classrooms, so it is reasonable to think that children are already honing their executive function and spatial skills when they use the materials in a less structured way (for a review of spatial thinking and how to incorporate it across the curriculum, see Newcombe & Frick 2010). However, many children, especially those from families with limited resources who may not have had many opportunities to play with materials like LEGOs or Play-Doh, tend to enter kindergarten with executive function and spatial skills that are not as well developed as their peers’ (Levine et al. 2012; Potter, Mashburn, & Grissmer 2013). These students may benefit from more structured design-copying activities—which fall in the category of guided object play—in conjunction with less structured, exploratory experiences (Weisberg, Hirsh-Pasek, & Golinkoff 2013; Cameron et al., forthcoming). Results from one study of Minds in Motion provide persuasive evidence that structured design-copying activities can improve executive function and spatial skills for children living in poverty (Brock et al. 2017); the research team’s analyses also suggest that these activities have the potential to improve math scores (Grissmer et al. 2013).
Although Minds in Motion is currently available only to teachers participating in an evaluation of the program, the principles underlying the curriculum can be incorporated with little preparation into most early childhood classrooms. Teachers usually have on hand many of the materials needed for design copying (such as crayons, felt pieces, blocks, and beads), so all they need to add is creative, colorful models for copying. Start with fairly simple models, and move on to more complex ones as children become more proficient. Consider dedicating a center to copying designs, and cycle interesting materials and models through it. And use and encourage spatial vocabulary—like above, below, beside, middle, and rotate—as you explain and guide children though each task; such language helps develop children’s spatial thinking, especially if children use the words too (Pruden, Levine, & Huttenlocher 2011).
Conclusion
Design copying may improve students’ mathematics abilities, but the most important message for teachers is not that they must have their students copy designs, but rather that activities that support math achievement do not always look like math. Design copying exercises and develops underlying cognitive skills—primarily executive function and spatial skills—that contribute to math ability. These cognitive skills provide the foundation for later learning in other subjects, as well. But this is not a new idea. Friedrich Froebel, the inventor of kindergarten, built his curriculum around presenting children with a sequence of so-called gifts, many of which are building materials to be used in activities quite similar to design copying. Echoes of Froebel’s methods are present in every block center and tub of pattern blocks. Modern psychological and educational research suggests that Froebel’s intuition to encourage young children to see, touch, and create may have been correct, at least as applied to math instruction. In the words of one of Froebel’s autobiographers, the “recognition and appreciation of form before nomenclature” was evident to him and now is equally evident to early childhood professionals (Brosterman 1997, 22).
References
American Academy of Pediatrics. 2009. Caring for Your Baby and Young Child: Birth to Age 5. 5th ed. New York: Bantam.
Best, J.R., & P.H. Miller. 2010. “A Developmental Perspective on Executive Function.” Child Development 81 (6): 1641–60.
Brock, L.L., W.M. Murrah, E.A. Cottone, A.J. Mashburn, & D.W. Grissmer. 2017. “An Afterschool Intervention Targeting Executive Function and Visuospatial Skills Also Improves Classroom Behavior.” International Journal of Behavioral Development.
Brosterman, N. 1997. Inventing Kindergarten. New York: Harry N. Abrams.
Bull, R., K.A. Espy, & S.A. Wiebe. 2008. “Short-Term Memory, Working Memory, and Executive Functioning in Preschoolers: Longitudinal Predictors of Mathematical Achievement at Age 7 Years.” Developmental Neuropsychology 33 (3): 205–28.
Cameron, C.E., L.L. Brock, W.M. Murrah, L.H. Bell, S.L. Worzalla, D.W. Grissmer, & F.J. Morrison. 2012. “Fine Motor Skills and Executive Function Both Contribute to Kindergarten Achievement.” Child Development 83 (4): 1229–44.
Cameron, C.E., L.L. Brock, W.M. Murrah, L.H. Bell, S.L. Worzalla, D.W. Grissmer, & F.J. Morrison. Forthcoming. Hands-On, Minds on: How Executive Function, Motor, and Spatial Skills Foster School Readiness. New York: Teachers College Press.
Carlson, A.G., E. Rowe, & T.W. Curby. 2013. “Disentangling Fine Motor Skills’ Relations to Academic Achievement: The Relative Contributions of Visual-Spatial Integration and Visual-Motor Coordination.” Journal of Genetic Psychology 174 (5–6): 514–33.
Chen, W.-B. 2010. “Fine Motor Skills As a Mediator of the Relationship between Parent-Child Interaction Quality and Child Cognitive Ability.” PhD dissertation, University of Virginia.
Dehaene, S. 2011. “The Number Sense: Fifteen Years Later.” Chap. 10 in The Number Sense: How the Mind Creates Mathematics. Rev. ed. New York: Oxford University Press.
Geary, D.C. 2013. “Early Foundations for Mathematics Learning and Their Relations to Learning Disabilities.” Current Directions in Psychological Science 22 (1): 23–27.
Grissmer, D.W., K.J. Grimm, S.M. Aiyer, W.M. Murrah, & J.S. Steele. 2010. “Fine Motor Skills and Early Comprehension of the World: Two New School Readiness Indicators.” Developmental Psychology 46 (5): 1008–17.
Grissmer, D.W., A. Mashburn, E. Cottone, L.L. Brock, W.M. Murrah, J. Blodgett, & C.E. Cameron. 2013. The Efficacy of Minds in Motion on Children’s Development of Executive Function, Visuo-Spatial and Math Skills. Paper presented at the Society for Research on Educational Effectiveness (SREE), Washington, DC. www.sree.org/conferences/2013f/program/downloads/abstracts/1003_4.pdf.
Levine, S.C., K.R. Ratliff, J. Huttenlocher, & J. Cannon. 2012. “Early Puzzle Play: A Predictor of Preschoolers’ Spatial Transformation Skill.” Developmental Psychology 48 (2): 530–42.
McClelland, M.M., & C.E. Cameron. 2012. “Self-Regulation in Early Childhood: Improving Conceptual Clarity and Developing Ecologically Valid Measures.” Child Development Perspectives 6 (2): 136–42.
Murrah, W.M. 2010. “Comparing Self-Regulatory and Early Academic Skills As Predictors of Later Math, Reading, and Science Elementary School Achievement.” Doctoral dissertation, University of Virginia..
Newcombe, N.S., & A. Frick. 2010. “Early Education for Spatial Intelligence: Why, What, and How.” Mind, Brain and Education 4 (3): 102–11.
Potter, D., A. Mashburn, & D.W. Grissmer. 2013. “The Family, Neuroscience, and Academic Skills: An Interdisciplinary Account of Social Class Gaps in Children’s Test Scores.” Social Science Research 42 (2): 446–64.
Pruden, S.M., S.C. Levine, & J. Huttenlocher. 2011. “Children’s Spatial Thinking: Does Talk about the Spatial World Matter?” Developmental Science 14 (6): 1417–30.
Uttal, D.H., N.G. Meadow, E. Tipton, L.L. Hand, A.R. Alden, C. Warren, & N.S. Newcombe. 2013. “The Malleability of Spatial Skills: A Meta-Analysis of Training Studies.” Psychological Bulletin 139 (2): 352–402.
Verdine, B.N., C.M. Irwin, R.M. Golinkoff, & K. Hirsh-Pasek 2014. “Contributions of Executive Function and Spatial Skills to Preschool Mathematics Achievement.” Journal of Experimental Child Psychology 126: 37–51.
Wai, J., D. Lubinski, & C.P. Benbow. 2009. “Spatial Ability for STEM Domains: Aligning over 50 Years of Cumulative Psychological Knowledge Solidifies Its Importance.” Journal of Educational Psychology 101 (4): 817–35.
Weisberg, D., K. Hirsh-Pasek, & R.M. Golinkoff. 2013. “Guided Play: Where Curricular Goals Meet a Playful Pedagogy.” Mind, Brain, and Education 7 (2): 104–12.
Photographs: © Getty Images; courtesy of the author
Anthony (Tony) I. Byers, PhD, is principal of the Graham and Parks Alternative Public School in Cambridge, Massachusetts. He has taught kindergarten and worked with the Foundations of Cognition and Learning (FOCAL) lab at the University of Virginia’s Center for Advanced Study of Teaching and Learning (CASTL).
Elizabeth (Beth) A. Cottone, PhD, has a background in psychology and special education. Beth has extensive experience in school leadership, and in clinical and after-school settings. She is the first author of the Minds in Motion curriculum.
Claire E. Cameron, PhD, is associate professor of early childhood in the Graduate School of Education at the University at Buffalo in New York. Claire studies cognitive development and assessment and is the author of Hands-On, Minds on: How Executive Function, Motor, and Spatial Skills Foster School Readiness. [email protected]